At Terraform Industries we believe in a future where energy is universally cheap, clean, and abundant. We’re developing a scalable electrolyzer to deliver the cheapest possible green hydrogen, which we use as a precursor chemical to make cheap synthetic carbon neutral natural gas in our Terraformer.

We find in our conversations with other builders in the climate/energy space that the destiny of hydrogen is often incompletely understood, so I’m writing this post to explain how Terraform is approaching the question.
Hydrogen production uses a lot of energy. Green hydrogen, produced without CO2 emissions, is currently a more expensive option than production from CH4, which produces a lot of CO2. We want green hydrogen to compete on cost, not just vibes. We believe that correctly developed energy should deliver a green dividend rather than demanding a green premium, and that rapid mass market adoption depends on beating the incumbent polluters on price.
(As an aside, it is quite obviously impossible to enforce universal adoption of more expensive carbon-free energy through, eg, mandatory carbon offsets, as higher gas prices are political suicide regardless of system of government.)
The cost of green hydrogen is a combination of the embodied energy (opex) and the amortized cost of the hydrogen electrolyzer (capex) which splits water into hydrogen and oxygen using electricity. Hydrogen distribution costs are also extremely high, but because Terraform is only using hydrogen as an immediate precursor we’re going to ignore distribution costs here. There are other methods for making carbon-free hydrogen, such as methane pyrolysis, which we will also not develop further in this post.
Let’s get quantitative. The best real world legacy electrolysis systems consume about 50 kWh/kg-H2. The theoretical optimum is 39.4 kWh/kg-H2, and Terraform’s electrolyzers are closer to 80 kWh/kg-H2 as it allows us to deliver a lower cost of hydrogen for reasons that will soon be apparent.
The cost goal for green hydrogen is $1/kg-H2. It is widely believed that if green hydrogen can reach this threshold it will be much much more attractive on the energy market. For context, the Inflation Reduction Act (IRA) green hydrogen production tax credit (PTC) in section 45V is worth $3/kg-H2, which may be enough to make it competitive with steam reforming in the short term.
The cheapest retail grid power is roughly $100/MWh. Using 50 kWh/kg-H2, we see that electricity cost alone works out at $5/kg-H2 – a clear non-starter. Assuming a $1/kg cost is achieved, electricity cost must be less than $20/MWh, and that’s assuming zero capex – an unwise assumption as electrolyzers are traditionally very expensive pieces of equipment.
Still, $20/MWh focuses the attention. Where can one find power that cheap or, ideally, cheaper? Enter solar! This isn’t the place to talk endlessly about solar, but if hypothetically we were looking for an energy generation technology which a) got 10x cheaper in the last 10 years, b) was scaling up production by at least 50% per year, and c) had no moving parts, solar photovoltaic (PV) is it! Indeed, in many large markets today it is now cheaper to install and operate a solar plant than to operate an already existing coal or gas plant. And if you love some other electricity generation technology, I’m happy for you, please reach $10/MWh LCOE and I’ll buy terawatts of it.
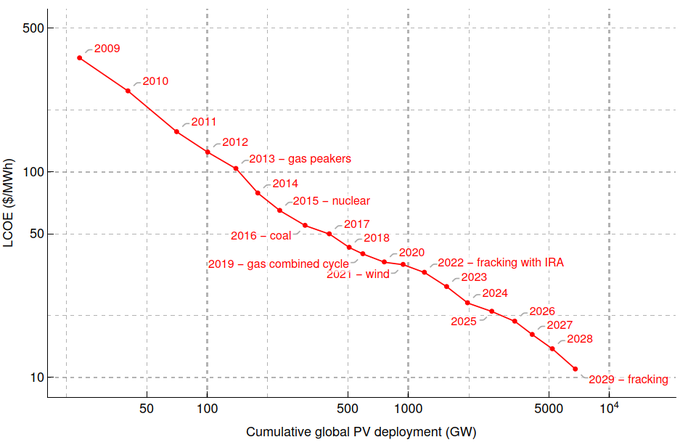
We want the cheapest possible power. What adds cost to solar power? Moving it from the array to another place. Using it at night. Using it when there are clouds blowing by. A typical solar-grid system today will have, in addition to the solar panels, a large and expensive “balance of plant” including inverters, battery storage, substations, grid interconnects, and then the rest of the electricity distribution grid, which includes long distance transmission lines, more substations, transformers, power lines, repair crews, insurance, fire safety, billing departments, legacy power plants, etc etc etc.
We want the cheapest possible power, so we have to delete all these additional cost sinks which do not actually make power and which add much much more than $20/MWh in cost. Then, determine if we can use the resulting, cheapest possible raw solar electricity to make hydrogen. Indeed, right now we have a test rig set up to derisk solar panels directly feeding an electrolyzer, without any intermediate power conversion.
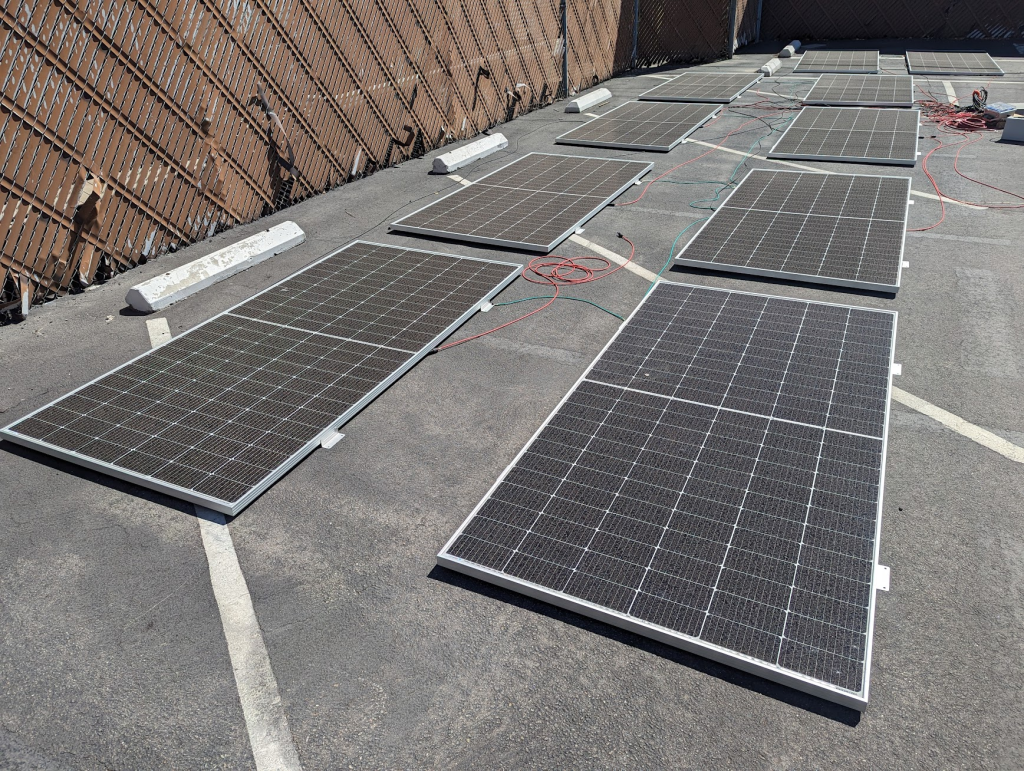
The single biggest objection we hear to feeding raw solar direct current (DC) into our electrolyzer is that solar utilization is around 0.25 so our electrolyzer will need to be able to throttle down overnight, and our electrolyzer utilization will only be 0.25. For a given quantity of hydrogen, we will need 4x as many electrolyzers and they are extremely expensive.
Adding batteries to smooth out supply adds substantial cost, with the cheapest geography-dependent wind/solar/battery green power coming in at about $45/MWh, or $2.25/kg-H2 on power alone. At current rates of battery cost improvement, it will be several decades until 24/7 power will be less than $1/kg-H2, so we must find another way!
Let’s detour slightly into electrolyzer cost land. Large scale 50 kWh/kg-H2 electrolyzers typically cost $1000s/kW, but we’ll stick to $1000/kW as a nice round number. 1 MWh of electricity produces 20 kg of H2. If we operate a $1m, 1 MW electrolyzer for 50,000 hours (5.7 years flat out), we will produce 1,000,000 kg-H2. Assuming zero financing or maintenance costs and total amortization over that period, capex alone works out to $1/kg, which is extremely ominous. I can’t see how we can get to $1/kg-H2 if capex is $1/kg and the cheapest 24/7 power is $2.25/kg-H2, for a total of $3.25/kg-H2.
50 kWh/kg-H2 is already close to ideal electrical efficiency, so the only way to cut the per-unit capex cost is to amortize equipment over a longer time period. But even if 0% financing and $0 maintenance costs can be found, hitting $1/kg-H2 will require (eg) 24/7 electricity for $15/MWh and a 25 year payback period, which places severe constraints on the industry’s ability to scale up quickly. There just isn’t that much 0% interest money on 25 year loans floating around.
Why are electrolyzers so expensive? To hit 50 kWh/kg-H2 or ~80% efficiency, electrolyzers need to use expensive platinum group metal electrodes, special separation membranes, heat exchangers, pumps, post-processing, and extremely high pressures and temperatures, which further restrict material choices and manufacturing processes. But in essence, electrolyzers are glorified electric resistors, with no more intrinsic complexity than a kettle that boils water and costs $15/kW, including free two day shipping from China!
Let’s re-examine the above hopeless economics with a different set of numbers. For the sake of argument, let’s say the Terraform electrolyzer can hit $100/kW and 80 kWh/kg-H2. This is much cheaper than a traditional electrolyzer, but it’s also just 50% efficient, which enables us to delete all the expensive stuff. Critically, it is happy to eat variable diurnal solar DC directly from the panel at $20/MWh. We’ll amortize over the same 5.7 years, but assume only 0.25 utilization for a total of 12500 hours.
Our hypothetical 1 MW electrolyzer costs just $100,000. Over 12,500 hours it consumes 12,500 MWh, producing 156,250 kg-H2, costing just $0.64/kg-H2 for capex. Power costs work out to $1.60/kg-H2, for a total of just $2.24/kg-H2 – more than $1 cheaper than the higher utilization, higher efficiency legacy electrolyzer model.
This is interesting – it goes against our intuition about the virtue of high efficiency and high capital utilization. But it’s not particularly mysterious. Hydrogen is an energy product, and if we’re doing it right then 70-80% of the product cost will be electricity opex. This is particularly important in the context of solar cost continuing to decline. A high capex option will still cost a lot even if electricity cost goes to zero, whereas a lower efficiency, higher power consumption option will pass cost savings on cheap power through to the consumer. Practically speaking, the best way to “short” future solar cost declines is to develop infrastructure now that achieves highly scalable low capex by trading away some electrical efficiency and being flexible enough to consume intermittent renewable electricity sources.
To make this future extremely legible, let’s run the numbers again but with $10/MWh solar cost, such as we expect by about 2028, the same lower efficiency (50%, 80 kWh/kg-H2) and with $50/kW capex, which is still more expensive than most household appliances of comparable complexity. Now capex is just $0.32/kg-H2 while opex is $0.80/kg-H2, for a total of just $1.12/kg-H2, which is extremely close to the magical $1/kg, while legacy systems that cannot exploit cheap solar will still be around $3/kg.
For the fully general case, the figures below give quantitative model analysis showing that the cost penalty of solar load shifting beyond ~0.25 utilization places insurmountable barriers on reaching $1/kg-H2 for green hydrogen. There is a saving grace! We can build low utilization lower efficiency electrolyzers cheaply enough to get to $1/kg-H2, but we have to follow the cheapest solar wherever it leads – in this case away from 100% utilization and high electrical efficiency.
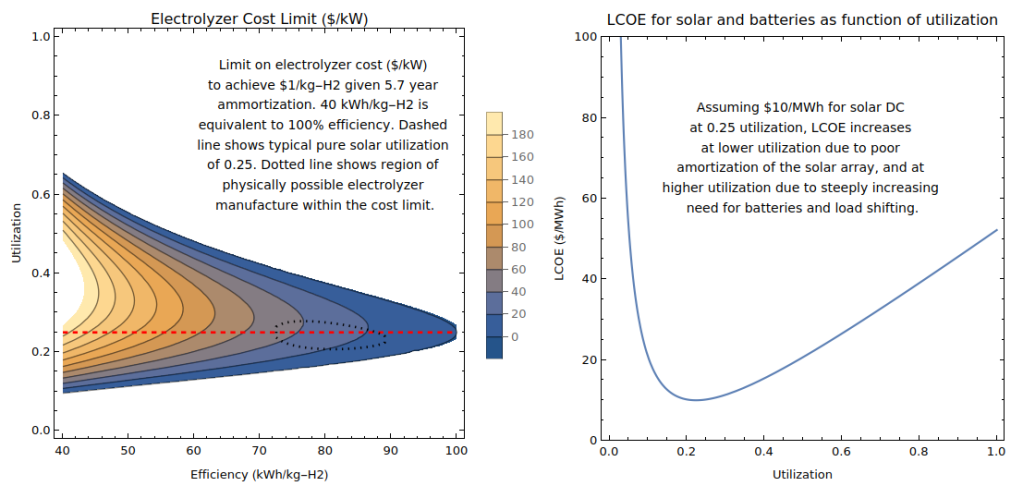
I am 100% confident that by the time we are mass producing this system at these prices later this decade, not only will this be the cheapest hydrogen available anywhere, it will also be the cheapest chemical energy and by far the most scalable – offering <5 year ROI with an extremely competitive supply chain and able to address nearly every market on Earth. This is in sharp contrast to fossil oil and gas, which is hidden underground in just a few places on Earth and impossible to exploit without global infrastructure.
Indeed, as we’ve written before once solar synthetic gas hits the cost parity threshold with fossil hydrocarbons there will be unstoppable global development until further price reductions and capacity increases saturate demand. Conservatively, we expect the oil and gas industry to grow from $6.4T/year today to at least $25T/year once second order global economic growth is factored in, and if we execute well we could be done by ~2040.
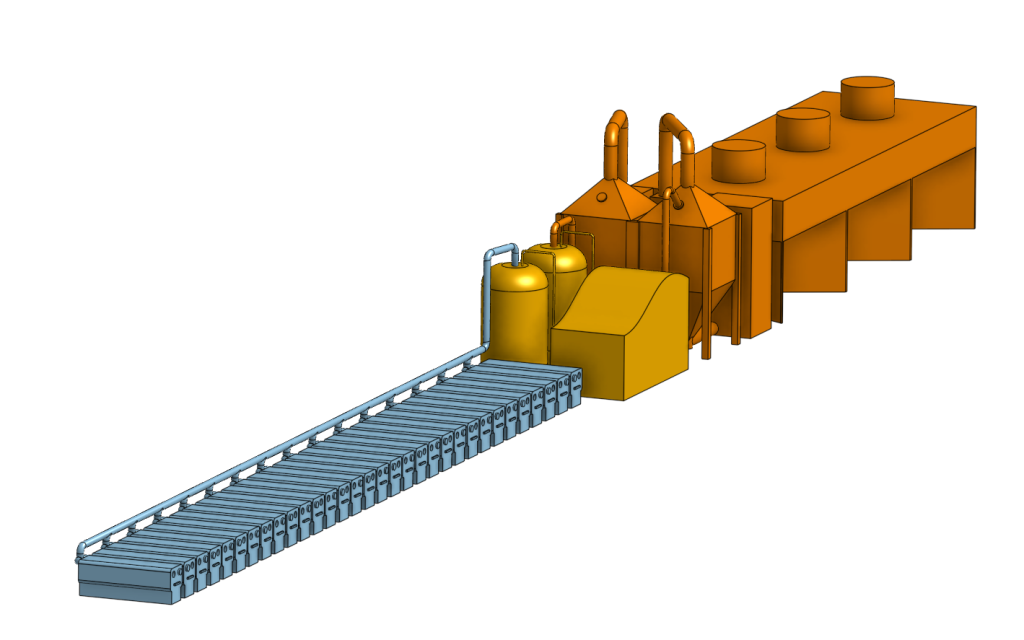
Render of 1 MW Terraformer showing electrolyzer stacks (blue), CO2 capture system (orange) and fuel synthesis reactor (yellow). There are 32 electrolyzer stacks each consuming 25 kW directly from adjacent panels (not pictured).
This natural implication of the basic underlying economics implies some potentially unexpected future developments, which I will list here for completeness. We expect to see the development of roughly 400 TW of solar worldwide, >90% of which will supply synthetic fuels most likely via hydrogen electrolysis and then catalytic synthesis using direct air capture (DAC) CO2. This will consume roughly 5% of Earth’s land surface area, comparable to current roads and urbanization and a lot less (in terms of both area and ecological impact) than current exploitation with farming and forestry, not to mention conventional fossil fuels extraction or uninhabited deserts.
While electrification will continue to displace existing hydrocarbon usage, particularly in ground transportation and some residential heating applications, we expect hydrocarbon consumption to shift and grow, particularly in manufacturing and aviation as we move towards a near term future of unconditional material abundance for everyone on Earth. Do we want a future where Europe pushes through mandatory low quality carbon offsets on airfares, further restricting the privilege of high speed travel to the richest of the rich? Or do we want a future where cheap carbon-neutral aviation fuel expands access to globe-spanning travel opportunities for hundreds of millions of people while also enabling a renaissance of supersonic transport?
While hydrocarbons today supply roughly 2/3 of the ~100 quads of energy consumed annually in the US, by ~2040 hydrocarbons will be downstream of cheap solar, just as nearly all grid electricity will be downstream of solar, providing energy to customers through parallel distribution networks of electricity, oil, and gas.
This Sankey diagram provides a rough sketch of the US energy economy as it existed in 2021.
This Sankey diagram shows how we expect natural economics forces will reshape the energy economy over the next two decades.
The Terraform electrolyzer is composed of roughly 4000 individual cells. 100% market saturation implies the deployment of 400 million 1 MW electrolyzers worldwide. Not including ongoing fleet turnover, we expect the synthetic fuel industry to build and deploy 1.6 trillion electrolyzer cells, each roughly the size and cost of a dining plate, over the next 20 years. On average this works out to 2500 cells per second! I am wary of exaggeration but I think it is fair to say that in terms of parts, factories, ramp rate, and energy consumption, this is the single largest technology roll out to occur in the history of humanity, and will cause the other so-called industrial revolutions to pale into insignificance.
We are hiring!
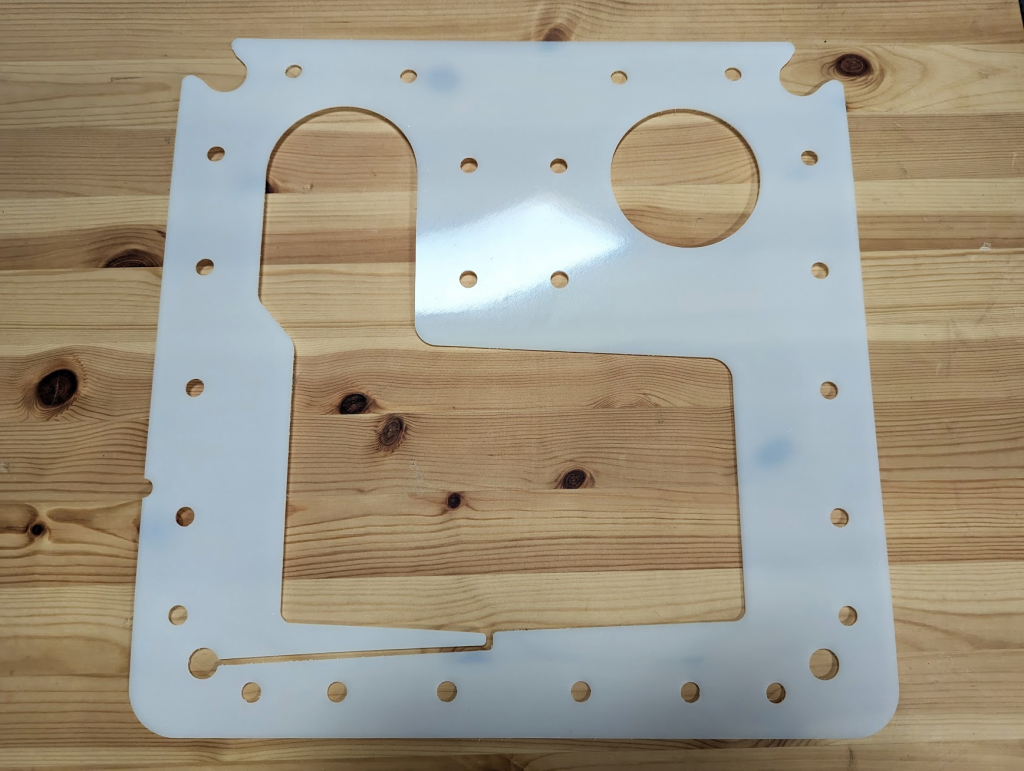
Here is a photo of part of our current electrolyzer design. If you have the vision, insight, and intuition to help us bring this electrolyzer to the masses then email us at hiring@terraformindustries.com. We are looking for a one-pager of constructively critical design review commentary demonstrating your understanding of its design constraints, requirements both real and unnecessary, and, of course, what we’ve missed.
Great! Now you just need to build out a hydrogen storage and use infrastructure that won’t blow up, and costs somewhere in the vicinity of using petroleum derivatives.
The point about using cheaper, nominally less efficient materials and techniques to achieve a better endpoint cost reminds me of SpaceX – they use RP1 instead of methane or liquid hydrogen for fuel, and they build out of stainless steel instead of using unobtainium, because the endpoint of overall cost per kg lifted is lower.
LikeLiked by 1 person
You’re kinda missing the point of the biz plan. There is **no** H2 storage: it’s immediately turned into methane and fed into the existing natural gas distribution system.
I have questions about how you build out natural gas distribution so that a bazillion 1MW generation units get access. One obvious way is to liquefy it and transport it by truck or rail, but the NIMBYs (even developing economy NIMBYs) are going to have something to say about that.
I’d like to see Casey address this. My intuition is that feeder pipeline costs could be pretty close to balance-of-plant and electrical feeder costs. The whole point is to avoid all the expensive infrastructure costs.
BTW, SpaceX uses RP-1/LOX with Li-Al rocket bodies for Falcon 9. Starship will use stainless steel, but it’s methane/LOX.
LikeLiked by 2 people
I like to view an overall resolution of the climate change problem in terms of four overlapping efforts:
1) Gasify: Replace coal with nat gas as quickly as possible. This is hopefully turning into a no-brainer, although beefing up–and cleaning up–the nat gas distribution system isn’t gonna be cheap. Timeframe: 2015-2040.
2) Decarbonize: Replace fossil-powered gasoline, diesel, and nat gas transportation with something carbon-neutral. Green methane, à la Terraformer, sounds like it plays well in the space, as do EVs and dispatchable renewable grid power (i.e., solar/wind farms backed up with enough storage to be reliable). Timeframe: Sorta now, but really more like 2030-2070, if we’re looking at anything of reasonable scale.
3) Mitigate. Since decarbonization doesn’t remove CO2 from the atmosphere and the overhang is almost certainly going to take us several tenths of a degree C above 1.5ºC compared to pre-industrial, we’re going to need some geoengineering. I don’t have a strong opinion on what the right tech is, but I **do** have a strong opinion that preparing the public for the necessity of doing this, and getting our technical ducks in a row, is crucially important, so that we can use this in the 2040-2140 timeframe.
4) Restore the atmosphere to pre-industrial GHG levels. IMO, carbon capture is still pretty much science fiction right now, but it won’t be forever. One thing that’s almost certainly true, however, is that it’s only affordable in a civilization that’s awash in cheap energy. Again, I don’t have a strong opinion on what science fiction energy technology is used, as long as one exists, and CC eventually becomes cheap and scalable. The good news is we don’t have to do this right away: a timeframe of 2070-2200 is probably fine, as long as it happens before something goes wrong with mitigation and we have a termination shock.
I like the idea of relying on green methane as a big chunk of the “decarbonize” part of the plan. I’m still skeptical of the scaling, and, as I mentioned up-thread, I’m skeptical that green methane distributes as cheaply as is being assumed. But driving fossil fuel extraction to zero seems like the necessary precondition for everything else. I hope a million flowers bloom, and then one tough, nasty flower grows all over everything in short order.
LikeLiked by 1 person
There is one important caveat to the Terraformer: how leaky is it?
The paper I read is: Ocko, I. B. and Hamburg, S. P.: Climate consequences of hydrogen emissions, Atmos. Chem. Phys., 22, 9349–9368, https://acp.copernicus.org/articles/22/9349/2022/, 2022.
Hydrogen is apparently a rather potent short-term greenhouse gas, and it is notorious for escaping when you don’t want it. Add in any methane leaks, and the environmental friendliness of stacking these to the rafters is reduced. Not eliminated; it’s still far better than the alternative (the paper’s authors estimate that even in their worst case of continuous emissions, there would be a 65% reduction in climate impact of implementing a full green hydrogen stack), but it certainly doesn’t look good if and when you meet any opposition.
I’m not in opposition. I want this to succeed.
LikeLike
Yep, but we can price leaks and slow them down enough.
LikeLike
Casey makes two errors, both in his favor.
First, solar doesn’t consume land. Solar can be placed on farm and pasture land with minimal, often negative reduction in yield. This will happen less in the US than many other places just because there is so damn much unused land, but might become important in northeastern states, as it must in Europe. Japan does it now, although some say the agricultural usage is just for show to claim tax credits.
Second, hydrogen production will not mainly depend on bespoke solar and wind farms. Every big, overprovisioned producer will drive hydrogen electrolysers to absorb production above immediate demand after storage is charged, and provide a second revenue stream. The effect is that power to drive the system will be free, or “zero marginal cost”. Producers that fail to direct overproduction to H2 production will be underbid by those that do.
Electrolysers driven only by overproduction will be used intermittently, making capital cost all-important in the way Casey describes.
LikeLike
You are missing some important opportunities concerning green hydrogen. I have talked to a facility in Southern California that —
1 – Uses Oxygen in their process. So, the byproduct of your electrolyzer – oxygen has a market. (Improves the economics).
2 – This facility has an offramp of a major freeway at their front gate. No hydrogen transportation costs.
3 – There are currently very significant amounts of solar and wind power that are being curtailed in California (thrown away – over supply, system operation & market structure problems). If you do not believe that – look at the California Independent System Operator web site. The problem is you must get that free power across the transmission system and to the customer side of the meter.
4 – You may not need the complexity of your process to make the economics work.
I would love to develop a test facility at this site and work with the regulatory and serving utility economics to make something happen that is actually green hydrogen vehicle fuel.
LikeLike
Hi Casey, this is a fantastic write up. The idea is in the scope of Amazon’s Sustainable Logistics Challenge, since it has the potential to accelerate the development of e-fuels. You can apply at the link below. Deadline is Sep 15.
LikeLike
The electrolyzer/solar part may work if your prediction about the cost of power is correct, but the free air CO2 capture maybe never will. Earth is not Mars or Venus, so you will never have a cheap stream of pure CO2 outside of gas separation from the oil and gas industry. Ask a tree–free air capture is hard! It is better to gasify biomass, since you can use the entire Earth for capture and the resulting CO/CO2/H2O is highly concentrated. Of course, if you do that, you are better off making biofuel!
Best to focus on the possible, with your cheap Hydrogen supplying our greatest users (and also leaving natural gas in the ground): ammonia production, crude refining, chemical production, and maybe storage for off-peak electricity, unless what you really want to get a contract with SpaceX for a fuel plant on Mars!
LikeLike
Cheap DAC is possible, it’s not even all that hard with cheap power.
LikeLike