I’ve been putting this one off but no longer. Let’s talk about geoengineering.
At Terraform Industries, we’re developing a carbon neutral supply chain for cheap, unlimited hydrocarbons derived only from sunlight and air. With this and similar technologies, I’m confident that humanity will move beyond fossil fuels as soon as 2040, and not a moment too soon.
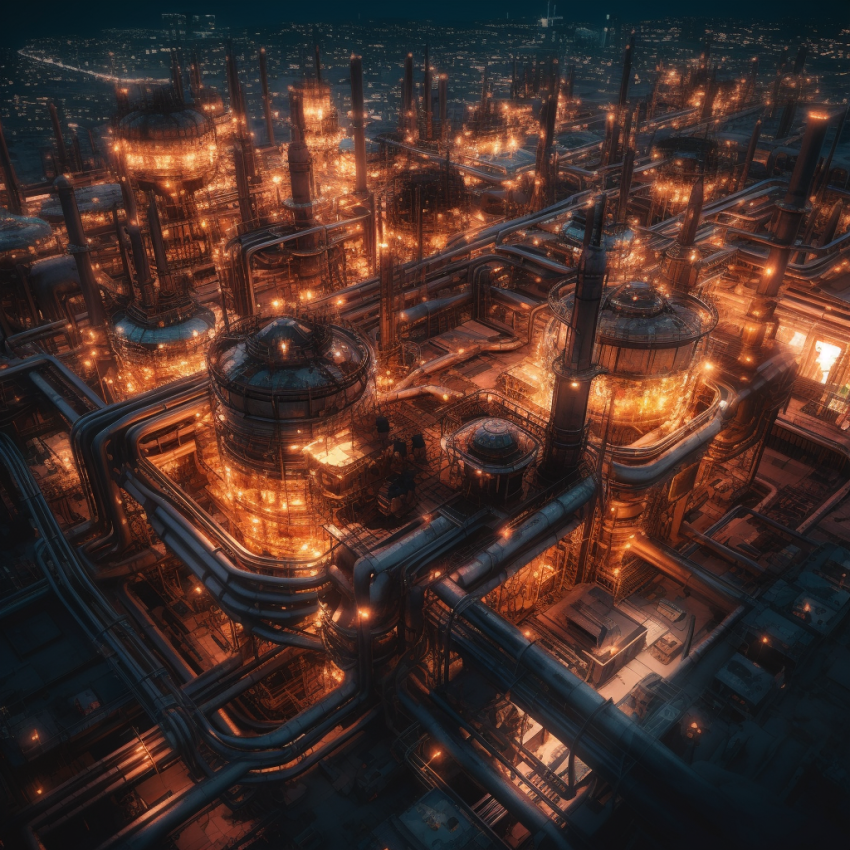
In other words, congratulations everyone, we did it! Between synthetic fuels and electrification, we’ve solved the climate challenge. There’s some implementation to be done but salvation no longer requires mass starvation or global totalitarianism or fusion-powered cell phones.
If only it were this simple. Yes, relatively soon we’ll slow down on putting new CO2 into the air, and plants eat up to 5 GT per year. But, we are still living beneath a sky with 2 teratons of excess CO2 emitted over the last 200 years, which will continue to warm the Earth for centuries to come. As the Earth warms, damage to the environment, ice caps, arctic greenhouse gas stores, and human lives will only intensify.
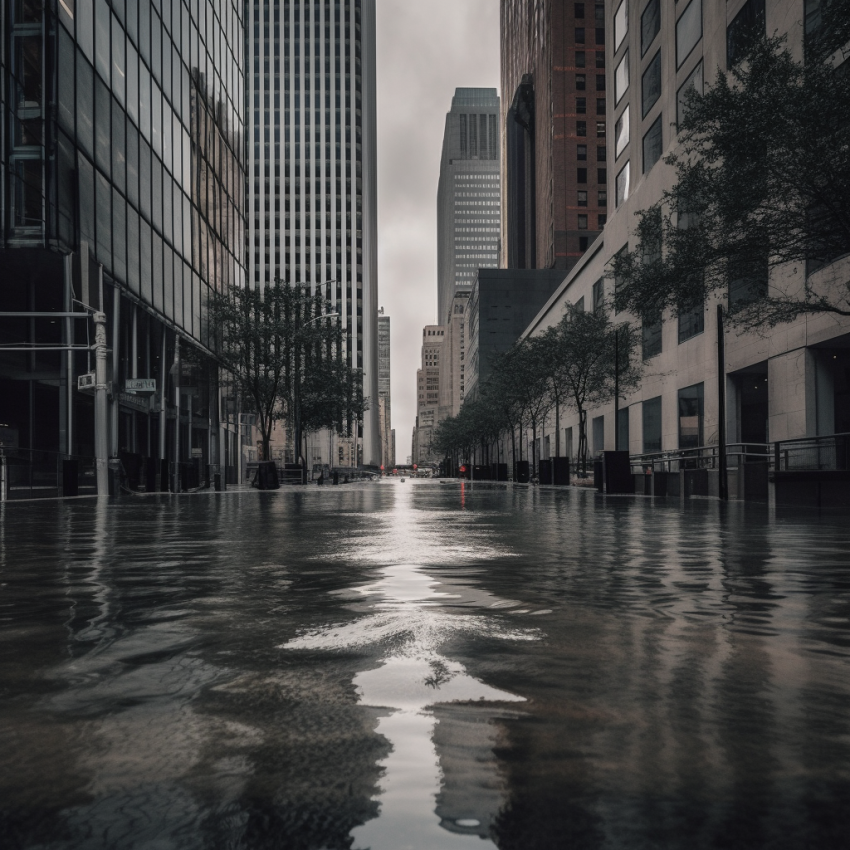
With synthetic fuel and electrification, the Earth may end up like a patient who made it to the OR only to bleed out because no-one applied a tourniquet when they had a chance.
Fortunately, we have a chance. Synthetic fuel takes care of new CO2 emission, and two specific kinds of geoengineering can take care of legacy warming in a way that safeguards our planet’s wellbeing for future generations and staunches the bleeding for the next couple of crucial decades while we get the job done.
The first task is to sop up excess legacy CO2. The solution here is cheap and straightforward. Accelerated weathering of mafic rocks increases the surface area available to absorb CO2. There are a bunch of ways of doing this, but the easiest and cheapest seems to be to grind up a couple of tropical volcanic mountains and sluice the resulting rock flour into the warm, shallow oceans. The rock dust floats around for a few weeks absorbing CO2 before sinking, permanently sequestering the CO2. In fact, oceanic carbonate rock (limestone, dolomite, etc) currently stores far more CO2, laid down over hundreds of millions of years by coral and other reef-building organisms, than exists in the atmosphere and oceans.
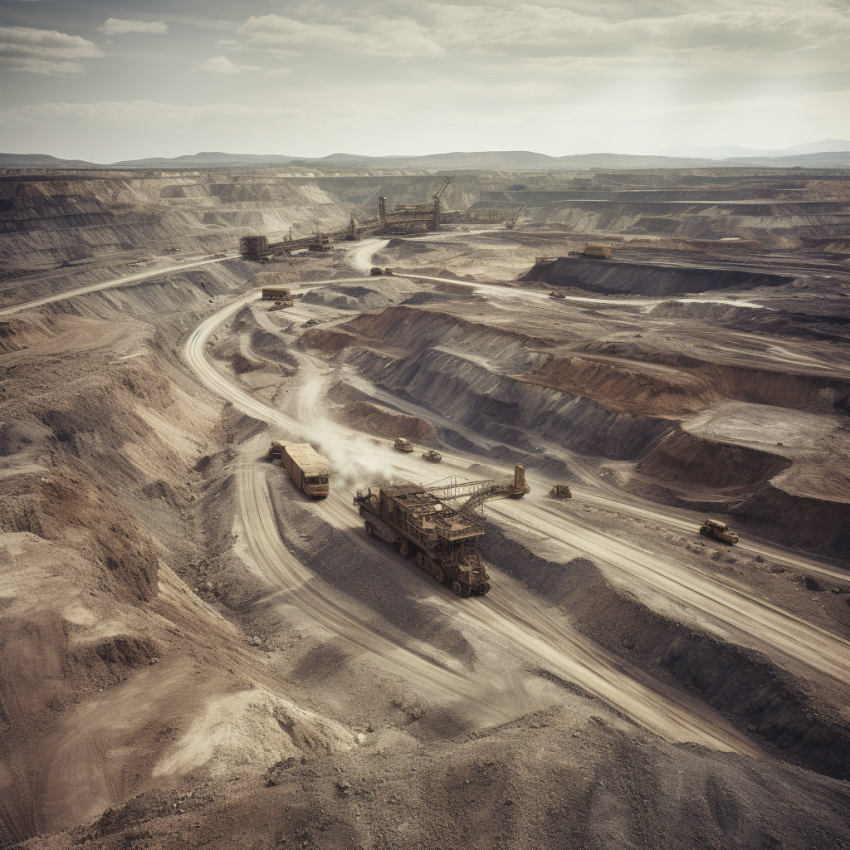
Campbell Nilsen wrote a great piece about this recently so I won’t go into vast detail. [And another as a follow up to this, on stratospheric particles.] Overall costs could drop below $20/T-CO2, which means we can soak up one of the two excess teratons of CO2, returning atmospheric CO2 to about 350 ppm, for about $400b/year over the next 40 years. Not cheap, but better than the alternative, and that’s while letting plants do 20% of the work. They could do all of it if we could wait 200 years, but we can’t. By then the Earth would have warmed beyond the point where we can sustain agriculture and trade, which means mass starvation. I’m going to go out on a limb here and say that avoidable mass starvation is a moral catastrophe and we should not do it.
So much for CO2. We’ll no longer extract it and we can sequester it for trivial cost and environmental impact compared to the inevitable outcome of doing nothing.
How do we keep the world cool for the next few decades while we upgrade our industry to a post-carbon world and scale up CO2 removal? It does us no good to be stable at 350 ppm by 2060 if we’ve already lost Greenland, the West Antarctic ice sheet, and 7 m + 4 m of coastline respectively. Of course neither ice sheet could float completely in just 40 years but our (in)actions in the next few decades could easily lock in 11 m of irreversible global sea level rise, to crush our descendants behind ever higher tides over the next century or so. Even if we successfully decarbonize and sequester excess CO2. And that doesn’t include other impacts of warmer weather, including famine, flood, fire, wet bulb mass fatalities, displacement, conflict…
What we need is a short term tourniquet to take the edge off global heating while we give the long term fixes time to work.
Fortunately, we have one and it’s safe, cheap, and effective. I’m talking about Solar Radiation Management. The problem with the CO2 we emit every day is that it sits in the atmosphere for hundreds of years and reduces the amount of heat the Earth is able to radiate. Not by much, but enough to warm the planet dangerously. In effect, it has made the planet slightly darker.
To compensate, we need some way to make the planet slightly lighter, to keep it in balance. In aggregate, the most reflective feature of the Earth is clouds, which reflect some of the sun’s light back into space. Relatively minuscule quantities of sulfur dioxide (SO2) in the stratosphere are extremely reflective, scattering some of the sun’s light before it hits the Earth, and in the process contributing to the atmospheric haze that gives sunsets their characteristic red color.
Stepping beyond the scolds, the gatekeepers, the fatalists and the “nyet” men, we’re going to have to do something like this if we don’t want to ruin the prospects of humanity for 100 generations, so now is the time to think about it.

You may have heard of stratospheric SO2 injection if you read “Ministry for the Future” by Kim Stanley Robinson or “Termination Shock” by Neal Stephenson, or followed what happened after the eruption of Mt Pinatubo in 1991, which lowered global temperatures by 0.5C for a year. While I’m looking forward to writing a side-by-side review of them both soon, in the real world, SO2 injection is a lot less dramatic than in either novel!

Stratospheric SO2 rains out of the atmosphere after a year or so, so unlike CO2 emissions it is relatively easy to control the dosing and the magnitude of the desired effect. SO2 injection can be ramped up or down as required on short timescales. As a rough rule of thumb, 1 g of stratospheric SO2 offsets the warming of 1 T of CO2 for 1 year.
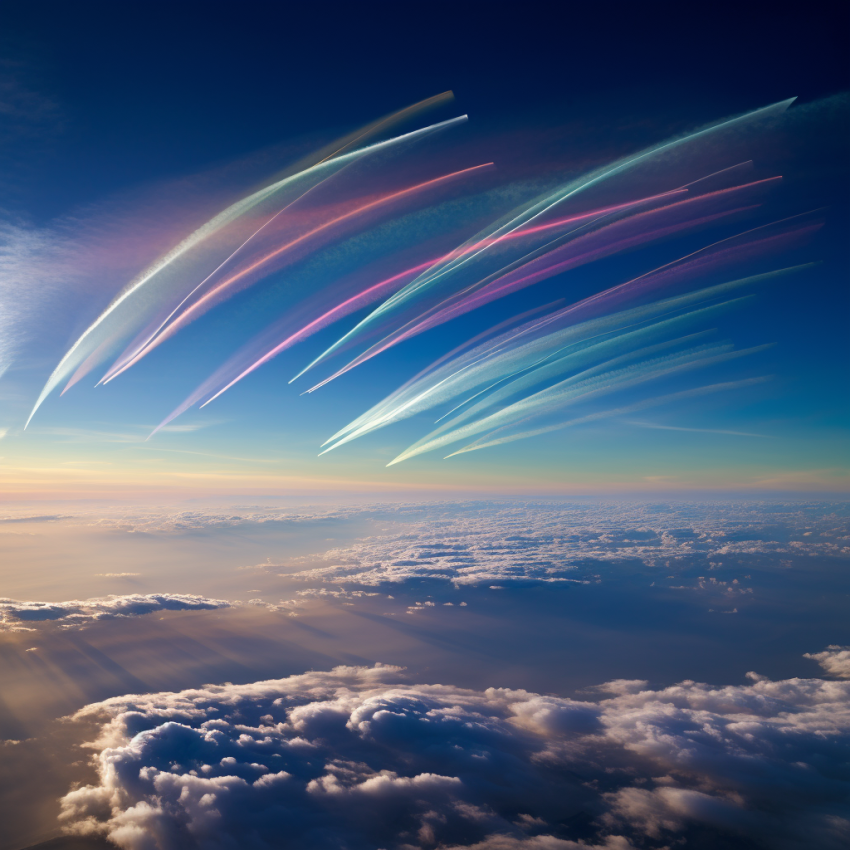
In fact, since the desulfurization of marine fuel over the last decade we’re seeing a substantial reduction in ground-level emission of SO2 and an increase in ocean heating in the North Atlantic and Pacific, potentially accounting for increased variability in rainfall on the eastern margins of both oceans. Ground level SO2 emission is regulated pollution, and for a good reason. It quickly falls out as sulfurous acid rain, harming the environment and not doing very much for cooling. SO2 stays in the stratosphere for much longer, so the relatively small quantities needed for cooling don’t cause concentrated acidic fallout as they would near, eg, a factory or refinery.
In “Termination Shock”, SO2 is delivered to the stratosphere using a giant gun in Texas (of course) while in “Ministry for the Future”, India unilaterally delivers it using a fleet of custom-built aircraft after 50 million people die in a wet bulb heat wave event. In fact, it’s much easier than this.
The start up Make Sunsets is delivering trivial quantities of SO2 to the atmosphere using weather balloons. Trivial, in the sense that for a trivial quantity of money, any of their customers (including me) can effectively offset the warming caused by their unavoidable CO2 emissions for a lifetime. Make Sunsets is showing that it is both legal and effective to tweak the emission profile of a single person to avoid global heating.
But if we’re doing this at global scale, we’re going to have to think bigger. Filling the largest practical single-use balloon with a lifting mixture of 2/3 hydrogen and 1/3 SO2, plus a tiny pressure-activated bursting mechanism, could get costs as low as 35c/kg.
1 kg of SO2 offsets 1000 T of CO2 for 1 year. With enhanced weathering, 1000 T of CO2 would cost at least $20k to deal with, and existing DAC+sequestration methods currently cost more like $1m. 35c! Now we’re talking.
A balloon composed of 20 micron LLDPE with a diameter of 10 m could be inflated as it is unrolled in a continuous process similar to the Sealed Air packaging system https://www.youtube.com/watch?v=tG92ac2YC0k. A balloon 1000 m long would weigh 500 kg and be filled to a depth of 50 m, containing ~5 T of gasses, of which 95% (by weight) is SO2 and the remainder is H2 to keep it buoyant.
As the balloon flies to 20 km or so, reducing atmospheric pressure causes steady inflation of the balloon until it is completely full. If the hydrogen mixture is burned to release the SO2, the LLDPE membrane will not return to the surface of the Earth.
Total cost is about $500 for the balloon, $300 for the H2, and $350 for the sulfur, which can be converted into SO2 by combustion. Adding 50% for overhead, we get $1700 per balloon or 35c/kg-SO2. One balloon offsets the warming of 350,000 Americans for one year. In other words, if just 1000 people in the US (and 10,000 worldwide) care enough to spend <$2000/year on launching the most giant, most awesome balloons to the stratosphere, we can offset CO2 heating effects until CO2 emissions and sequestration are under control. If they have a modest sailboat, they could even release the balloons without anyone even knowing about it.
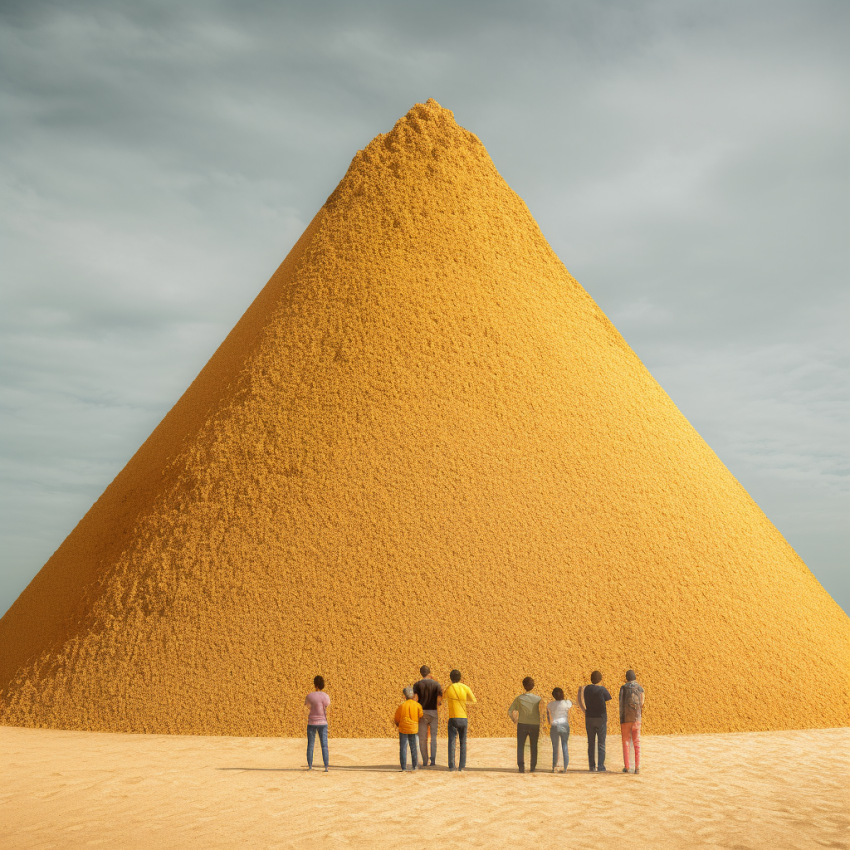
Indeed, if we want to offset the heat of 1 teraton of CO2, we need to launch 1 million tonnes of SO2 per year, costing just $350m/year. This is about 5% of the US’ annual production of sulfur. This costs less than 0.1% on an annual basis of the 40 year program to sequester a trillion tonnes of CO2. Just 200,000 of the 5 T balloons would be required annually, though in principle they could be made almost any size and released almost anywhere.
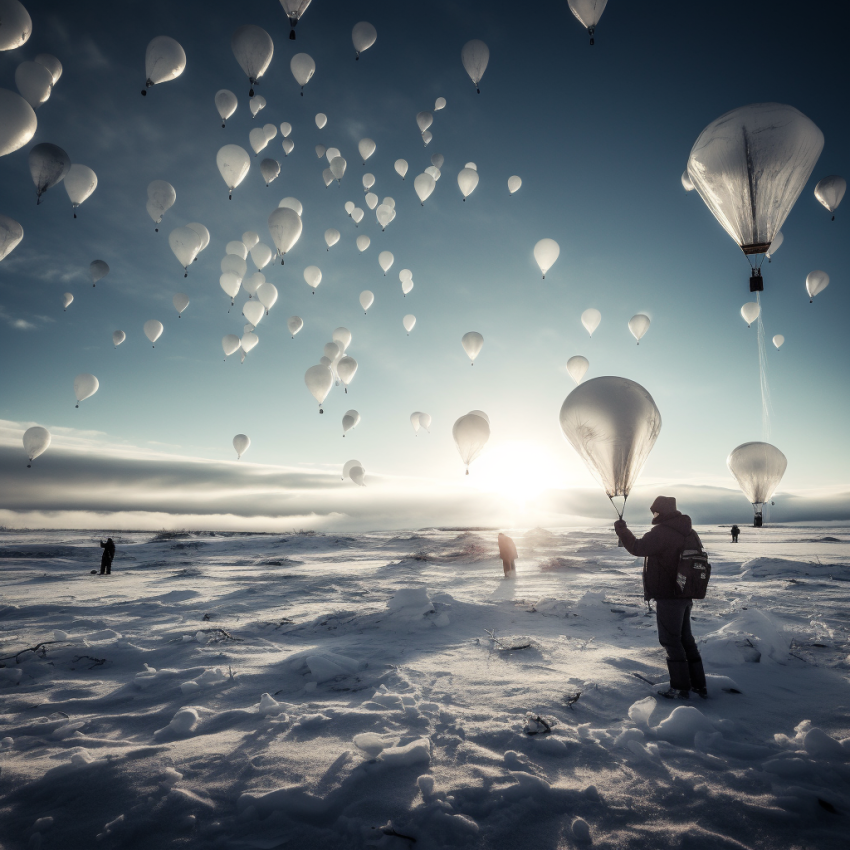
So how safe is it to put large quantities of SO2 in the atmosphere?
LikeLike
We’d be putting in less than we are now, and further from places it causes damage.
LikeLike
Awesome concept. I wonder if, in addition, mixing in some carbon sequestering powdered material could 1) provide some shade, as you mention at the start and 2) continue absorbing CO2 as it falls to earth.
Is anyone doing what you describe today?
Thanks
LikeLiked by 1 person
Make Sunsets is a startup in Silicon Valley that is already releasing SO2 into the stratosphere and working out the scaling problems to do it in bigger amounts. You can learn about it at makesunsets.com
LikeLike
Is the sulfur dropping back down from the stratosphere diffuse enough to avoid acid rain damage? How about calcium carbonate?
LikeLike
Yes, both.
LikeLike
Love it. 2 q’s
‘As the balloon flies to 20 km or so, reducing atmospheric pressure causes steady inflation of the balloon until it is completely full. If the hydrogen mixture is burned to release the SO2, the LLDPE membrane will not return to the surface of the Earth.’ – so the baloon itself persists indefinitely in the stratosphere, or the membrane gets burnt up somehow? if the baloon itself persists, how do you model that the cooling effect will be the same with all the SO2 concentrated in one spot vs spread out throughout the stratosphere?
Secondly, and maybe more observation than question – most of the other work on this, David Keith etc (which I’m not that familiar with) seems to suggest it’s much harder and more expensive than this – when this is one of the biggest problems we have, why aren’t we doing it already? costs of warming already well exceed the costs of this program.
LikeLike
It burns.
LikeLike
Very interesting „patch“ for the problem. Especially if the 35c thing works.
But for „personal“ usage Id like to point out that this is a compounding effect right?
At the current rate of 10$/T and my assumption that we don’t get to remove C02 for a long time… for me to be and stay carbon neutral it is this year 100$ and in 10 years 1000$/year
LikeLike
This is correct, but hopefully your CO2 footprint shrinks over time as renewable energy is more accessible and less expensive than fossil fuels. Also, if you commit to a monthly subscription, the price per Cooling Credit drops down to $1 vs. $10 for a one-off purchase. Here’s a link: https://buy.stripe.com/4gw14BfV3elc8Cs3cd
LikeLike
Make Sunsets uses helium. Isn’t that a bad idea? I guess it doesn’t really matter at their current scale.
LikeLike
In future, we’ll have to use hydrogen.
LikeLike
Don’t forget to account for the global warming effect of the stratospheric water vapor from burning that hydrogen, which stays in the stratosphere much longer than it does in the troposphere. Also I believe there are concerns that both hydrogen and sulphur dioxide can affect the chemistry of the ozone layer.
The other problem is even if you get the average temperature you want, the climate still changes, because solar radiation management cools differently from how the greenhouse gases warm. SRM only has an effect during the daytime, and disproportionately during summer, while GHGs block outgoing radiation throughout the day/year. A balanced CO2+SO2 increase will cool the tropics while still warming the poles, and generally reduce rainfall.
What is needed are more fine grained geoengineering approaches. I’m not sure if cloud brightening can be done in a regional rather than global way. I’ve read that it may be possible to inject SO2 at higher latitudes and have it stay there so that the cooling effect is mainly over the poles. I’d feel better about doing that, though you might need to use more sulphur to get the same overall effect (or maybe not thanks to ice sheet feedback).
LikeLike
No one talks about it, but hypothetically we could air-condition the planet.
When you run a normal-sized air conditioner, it dumps the heat outside your window, or outside the whole building. Either way, the hot air just mixes with the surrounding air and warms up the air at ground level. If you run a factory, doing some energy-intensive process at industrial scale, the steam vented out your smokestack can rise a few hundred feet into the air in the time it takes to mix with the surrounding air. If you ran a really big air conditioner, there would be enough heat released that it would rise to the tropopause, above most of the greenhouse gases.
The most powerful greenhouse gas is water vapor. It doesn’t count as a forcing, because its concentration distribution through the atmosphere is an endogenous variable. Rising air is normally saturated, because it has usually come from the surface where the temperature is higher and there’s water that can evaporate; sinking air is unsaturated (when it’s not inside a cloud), because when it was higher any water above the saturation vapor pressure for the colder temperature there precipitated out. The total amount of rising air and sinking air has to balance, but not the area. You can have a small volume of air rising quickly, balanced by a large volume of air sinking slowly. Tall thunderstorms are small (compared to the size of the planet) areas of fast-rising air. Anything that creates more tall thunderstorms should lower the average humidity of the atmosphere and thus cool the planet.
LikeLike
This is true, and in fact cyclones/hurricanes are gigantic heat engines that pump heat from the ocean into space with 99.99% Carnot efficiency. We are warming the ocean and they are getting more intense, which is sub-optimal for coastal areas.
LikeLike
At the linked page advocating grinding olivine and dumping it in shallow areas of ocean, the author takes for granted that energy cost will be much higher than capital cost, to the point where capital cost is basically negligible. It seems to me that this is almost necessarily incorrect.
The price of energy can literally be less than zero, if you’re taking energy out only when the grid is overloaded by variation in the output of wind and solar. Realistically, the cost of energy can be extremely low when those variable sources are producing excess electricity; up to some point, building excess capacity is cheaper than building storage capacity. If capital cost is low enough, you can design your system to run only when that excess is available.
I’m inclined to guess that sand is a better particle size. The energy cost is roughly proportional to the amount of area exposed: processing a larger amount of rock into sand is comparable in cost per ton of CO2 to processing a smaller amount into silt. So you want the option that’s better for disposal. Sand normally circulates along beaches under the action of waves and tides. Silt spreads out farther from shore and blankets the bottom, deep enough to be beyond the reach of waves. Adding a hundred million tons of sand to the shoreline at a particular processing site would probably be less disruptive than adding one million tons of silt, or whatever it comes out to for the same CO2 removal.
LikeLiked by 1 person
If you have a great sand-production machine, the world will beat a path to your door, and you can solve some other ecological problems at the same time:
https://www.theguardian.com/global/2018/jul/01/riddle-of-the-sands-the-truth-behind-stolen-beaches-and-dredged-islands
LikeLiked by 1 person
Original author of the olivine piece here. The two major reasons why silt is to be preferred to sand are weathering rate and settling velocity.
I think I give an overview of weathering rate in the original article. Although the data has some fairly wide error bars, Hangx and Spiers’ article gave an average-time-to-total-weathering of 23 years for an olivine grain at 10 microns, vs. 700 years for 300 microns (regular sand size). I’d prefer not to wait until the late 3rd millennium to get global warming under control, so we’ll have to go smaller than sand.
Now for settling velocity, which I didn’t mention much in the original piece, but which was brought to my attention by a feature on Vesta published yesterday in *Nature*. Olivine is 26% denser than quartz, so even if you put a layer of olivine sand on top of regular sand, it’s going to sink faster than the quartz every time a storm blows through and roils the beach around. This is precisely what’s happened to Vesta: their olivine sand has been buried under a layer of quartz sand, where it’s not weathering even at the slow rates you’d get given the particle size. (They don’t say what their average particle size is, but I’m guessing at least 200 microns.)
The standard equation for settling velocity can be found on Wikipedia (https://en.wikipedia.org/wiki/Sediment_transport#Settling_velocity). I spent a few hours yesterday hashing out the relative settling velocities of quartz and olivine at various diameters. But, e.g., if you have quartz at 200 microns and olivine at 10 microns, in standard seawater at 25ºC, the quartz will settle about 170 times faster than the olivine, keeping the olivine on the surface.
Project Vesta is surely aware of this; I think the reason they’re not making silt is that their main experimental location is in the Hamptons, so sand-dumping is fine but silt-dumping isn’t.
LikeLike
Either way you need the same surface area of rock to absorb CO2, so bigger particles mean more rock.
Power cost is important, IIRC you need many gigawatts equivalent to run grinders, cutters, etc, which almost certainly means a dedicated power plant.
LikeLike
Glad to see CJH defend SO2 as a global cooling option. This option has taken a lot of heat as CO2 reduction is seen by some as the only virtuous path (not that many of those folks are living a low CO2 life). As CJH points out, even a small nation could mount a significant cooling effort with a small investment. This could also be done privately in international waters. Time to try and data collect.
LikeLike
I’m curious: Is there any reason to specifically use S02, besides the fact that it’s what volcanoes inject into the upper atmosphere, leading people to realize this approach to geoengineering might work? Isn’t it possible that some other substance might make more sense, from a standpoint of over-all benefit?
Silica fume might be less acidic. Ultra-fine iron oxide would also fertilize the oceans.
Have people gamed out other substances, or did they just fixate on SO2?
LikeLiked by 1 person
How about olivine?
LikeLike
SO2 is the the most well studied/researched compound and as you pointed out, volcanos have shown it works as described without catastrophic effects. Calcium carbonate is another , but modeling is not great, and real-world effects are unclear. A study indicates it’s worse for the ozone layer. https://academiccommons.columbia.edu/doi/10.7916/d8-pw62-mc98
Diamond dust is another candidate, but extremely expensive.
LikeLike
I believe calcium carbonate has been floated as a possibility, and doesn’t come with the acid rain risk. Titanium dioxide also suggests itself–it’s pretty chemically inert and has a very high albedo, but it’s dense (4.23 g/cm³ vs. 2.71 /cm³ for CaCOɜ and 1.46 g/cm³ for liquid sulfur dioxide), so it might have to be renewed more regularly.
Casey, what is the formula for the sulfur dioxide rule of thumb–? if 1 gram of SO₂ with an albedo of X offsets the warming of one tonne of CO₂ for one year, then given a substance with an albedo of Y (that falls out of the atmosphere after such and such a period), how much warming does it counteract?
LikeLike
TiO2 is even better, but also more expensive.
LikeLike
How about calcium carbonate?
LikeLike